| |
Approximate magnitudes in some typical plasmas
Plasma Type |
ne cm-3 |
Te eV |
wpe sec-1 |
lD cm |
ne lD3 |
nenei sec-1 |
Interstellar gas |
1 |
1 |
6 ´ 104 |
7 ´ 102 |
4 ´ 108 |
7 ´ 10-5 |
Gaseous nebula |
103 |
1 |
2 ´ 106 |
20 |
107 |
6 ´ 10-2 |
Solar Corona |
109 |
102 |
2 ´ 109 |
2 ´ 10-1 |
8 ´ 106 |
60 |
Diffuse hot plasma |
1012 |
102 |
6 ´ 1010 |
7 ´ 10-3 |
4 ´ 105 |
40 |
Solar atmosphere, gas discharge |
1014 |
1 |
6 ´ 1011 |
7 ´ 10-5 |
40 |
2 ´ 109 |
Warm plasma |
1014 |
10 |
6 ´ 1011 |
2 ´ 10-4 |
103 |
107 |
Hot plasma |
1014 |
102 |
6 ´ 1011 |
7 ´ 10-4 |
4 ´ 104 |
4 ´ 106 |
Thermonuclear plasma |
1015 |
104 |
2 ´ 1012 |
2 ´ 10-3 |
107 |
5 ´ 104 |
Theta pinch |
1016 |
102 |
6 ´ 1012 |
7 ´ 10-5 |
4 ´ 103 |
3 ´ 108 |
Dense hot plasma |
1018 |
102 |
6 ´ 1013 |
7 ´ 10-6 |
4 ´ 102 |
2 ´ 1010 |
Laser plasma |
1020 |
102 |
6 ´ 1014 |
7 ´ 10-7 |
40 |
2 ´ 1012 |
electron density |
ne |
electron temperature |
Te |
electron plasma frequency |
wpe =
(4pnee2 / me)1/2 = 5.64 ´ 104 ne1/2
rad/sec
|
Debye length |
lD =
(kTe / 4pnee2)1/2 = 7.43 ´ 102 Te1/2 ne1/2 cm
|
electron collision rate |
nei =
2.91 ´ 10-6 ne ln L Te-3/2 sec-1
|
from page
40 of NRL Plasma Formulary
(see diagram on page
41 and LANL site diagram
from P-24 Plasma Physics Group in the Physics Division Annual Report, 1994)
Cool circumstellar dust and gas is constantly accumulating around a star which
is rapidly ejecting plasma; the rapid cooling of the plasma when it encounters
this
shell
can significantly enhance the non-equilibrium effects of
adiabatic expansion. Gas contact is so effective at producing rapid cooling that
Oda et al. (1987) have successfully operated an extreme ultraviolet plasma laser
using this mechanism alone, without the slightest amount of expansion :
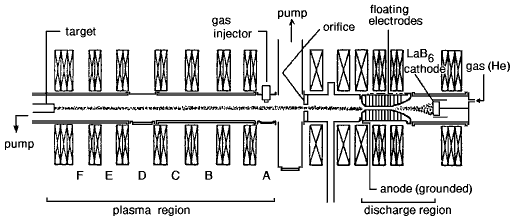 |
Gas-Contact Cooling Plasma Laser (TPD-I) : Magnetically confined stationary
helium plasma lasing in XUV 164 nm when the column is cooled by hydrogen gas
-contact. (Institute of Plasma Physics (IPP) Nagoya, Japan). |
Another advantage in stellar atmospheres are the typically very large distance
scales, a small population inversion produces radiation whose intensity would
exponentially grow in amplitude over the large distances to the point where it
dominates the spectrum with a strong broad emission line.
The strongest manifestation of natural lasers occur in
quasars.
Another disadvantage of laboratory plasma lasers is erosion damage.
'IT IS ALL DONE WITH MIRRORS'
In laboratory plasma lasers the lasing medium is of necessity limited
to small scales compared to astrophysical lasers. This drawback is partly
compensated by the advantage that we can place mirrors at both ends of the
lasing medium to produce a laser which seems to be much longer in
effective 'virtual' extent. By repeatedly bouncing off the mirrors the photons
make many back and forth passes, effectively lengthening the lasing medium.
Place yourself between a pair of parallel mirrors and see infinitely repeated
images of yourself, until you can no longer see the images due to the greenish
absorption of the glass and finite reflectivity of the mirrors.
Placing the lasing medium within a pair of mirrors also produces a
Fabry-Perot
resonant cavity whose spectral response is equivalent is the Airy function
consisting of a sequence of cavity 'modes'. Each mode correspond to an
electromagnetic standing wave which can occupy the cavity. With careful design,
the laser can be made to oscillate in only one of these modes which results in
a highly monochromatic collimated beam with extremely sharp spectral response.
NATURALLY OCCURRING, FUSION POWERED PLASMA LASERS
The maximum number of passes than a photon can make within a mirrored laboratory
laser cavity is limited by absorption and upper level depletion, which is
ultimately limited by the finite amount of energy pumped into the population
inversion. The shorter the wavelength the faster the energy must be pumped into
the upper levels. Although there are no mirrors in outer space, no such power
restrictions occur in astrophysical plasma lasers, which are pumped by an
enormously powerful fusion furnace.
AMPLIFIED SPONTANEOUS EMISSION
Even though most commercial lasers are designed to operate within a cavity and
produce very sharp emission lines; this is not necessarily so for lasers without
mirrors, especially in astrophysical environments where usually the spectral
line is broadened by Doppler effects, turbulence and rapid plasma ejections.
The stimulated emission occurs from many spontaneous emissions 'seeds' simultaneously,
i.e. amplified spontaneous emission; a technical name for the phenomena of
cooperative photon emission in the absence of mirrors.
Since the amplification is exponential over distance the gain is strongest along
an axis through which the longest path through the plasma can be attained.
If the plasma is very elongated the emission occurs almost exclusively along
that axis.
100 kilowatt Homebuilt Air Laser : pumped by electrical gas discharge,
it produces coherent 337 nm UV radiation from a molecular nitrogen transition.
There are no mirrors so the laser emits amplified spontaneous emission both
in the forward and backward direction simultaneously.
(Scientific American June 1974)
PRACTICAL USES OF PLASMA LASERS
HIGH-POWER, HIGH-EFFICIENCY LASERS
The simple fact the lasing medium is a highly ionized plasma
readily lends itself to virtually unlimited power amplification.
High Power
Continuous high power lasing is possible by using the geometry of a plasma
expanding away from a nozzle; since the decay of different parts of
the plasma occur at different times the active lasing portion of the jet
is constantly being replenished while simultaneously the depleted lasant
is rapidly carried out of the active zone.
This dual combination are two crucial ingredient in most high power lasers,
- Rapid overpopulation of the upper quantum level relative to the lower
level.
- Rapid de-population of the lower lasing level by transport of depleted
plasma out of the active lasing medium.
High Efficiency
High power can be achieved simultaneously with high efficiency by a suitable
choice of initial plasma parameters before the rapid cooling takes place :
In certain temperature ranges the thermodynamic population of certain ion
species is almost exclusively dominated by the ion consisting of a closed
electron shell. The ionization energy of an inert-gas like electron
configuration is much larger than the energy required to ionize less stable
configurations. The inert gas-like stage of ionization persists over
a much larger range of temperatures, and for certain particular temperatures
the competing ionic stages have negligible concentrations.
During the rapid cooling stage, almost all of these stable 'parent' ions within
the plasma contribute to the rapid three-body recombination of the free-electrons
into the upper laser level. This means that every ion in the plasma can
potentially contribute a stimulated photon to the amplification process.
The extent to which the excited ions can coherently amplify the light is
limited only by unwanted spontaneous emissions, and the requirement of
maintaining a suitable inversion rate for the specific ratio of stimulated
emission versus spontaneous emissions that the particular application requires.
The potential for high efficiency, unlimited power lasers is enormous, and
its practical uses in industry are too numerous to mention here.
REFERENCES
- Oda,T. et al.: 1987, Short Wavelength Laser and Their Applications,
Springer Verlag.
- Science on High-Energy Lasers: From Today to the NIF
|